Consider a turbojet powered airplane flying at a standard altitude of 20,000 ft at a velocity of 600 fps. The turbojet engine itself has inlet and exit areas of 8 and 5 ft2, respectively. The velocity and pressure of the exhaust gas at the exit are 1500 ft/s and 1240 lb/ft2, respectively. Calculate the thrust.
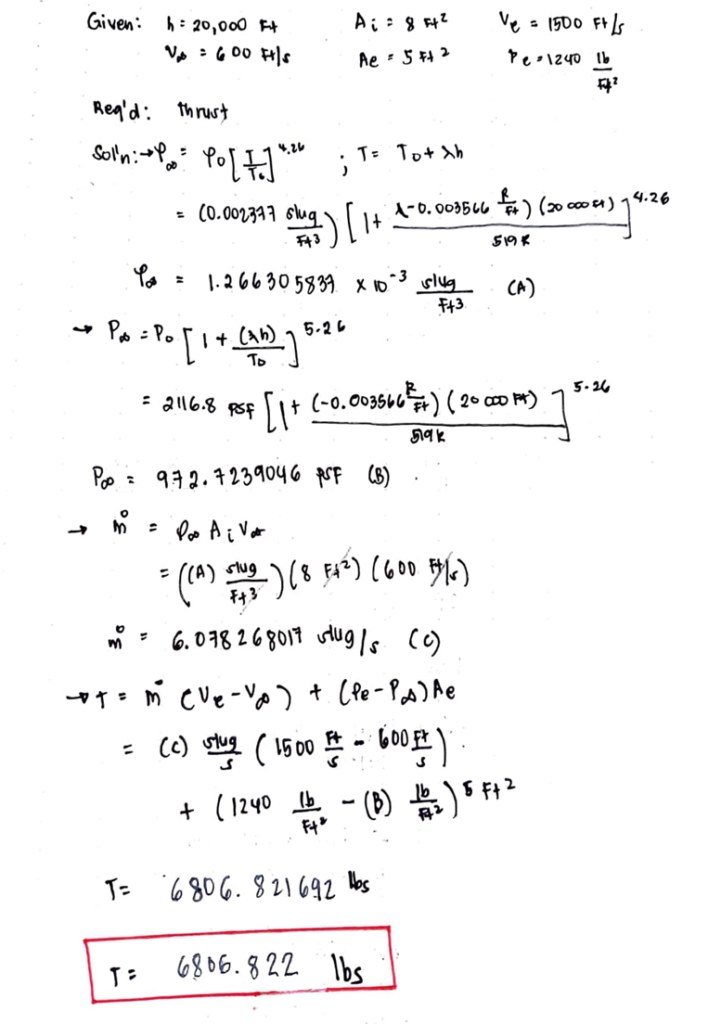
Consider a turbojet powered airplane flying at a standard altitude of 28,000 ft at a velocity of 650fps. The turbojet engine itself has inlet and exit areas of 6 and 4 ft2, respectively. The velocity and pressure of the exhaust gas at the exit are 1200 ft/s and 700 lb/ft2, respectively. Calculate the thrust of the turbojet.
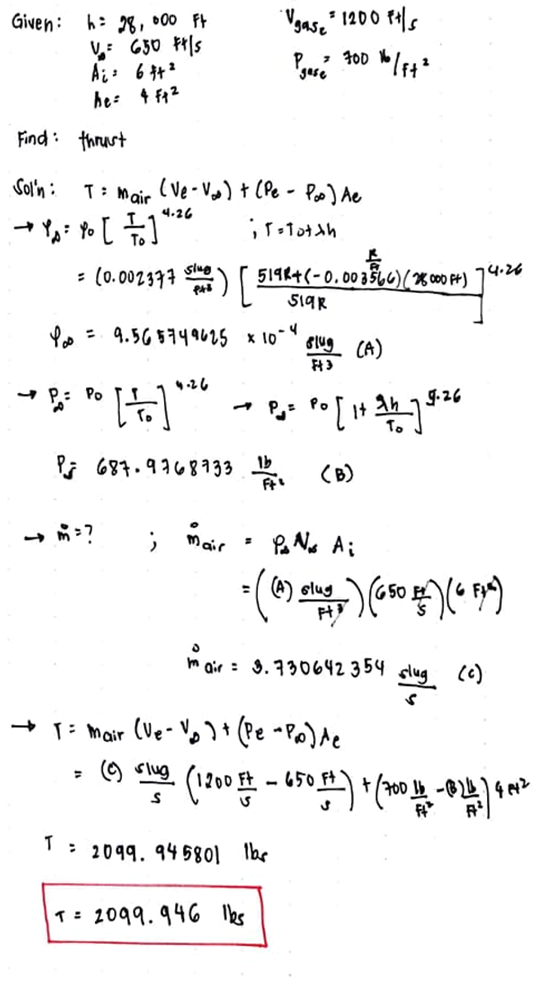
Calculate the brake horsepower for an engine that develops 600 foot-pounds of torque while turning at 2,700 rpm.

Calculate the indicated horsepower for a six-cylinder engine with a bore of five inches, a stroke of five inches, turning at 2,750 rpm, and with an IMEP of 125 psi per cylinder.

An airplane weighing 5000 lb is flying at standard sea level with a velocity of 200 mi/h. At this velocity, the L/D ratio is a maximum. The wing area and aspect ratio are 200 ft.2 and 8.5, respectively. The Oswald efficiency factor is 0.93. Calculate the total drag on the airplane.

Consider an airplane patterned after the Fairchild Republic A-10, a twin-jet attack aircraft. The airplane has the following characteristics: wing area = 47 m2, aspect ratio = 6.5, Oswald efficiency factor = 0.87, weight = 103,047 N, and parasite drag coefficient =0.032. The airplane is equipped with two jet engines with 40,298 N of static thrusts each at sea level.
Calculate and sketch the power required curve at sea level.
Calculate the maximum velocity at sea level.
Calculate and plot the power required curve at 5 km altitude.
Calculate the maximum velocity at 5 km altitude.





- Consider an airplane patterned after the Beechcraft Bonanza V-tailed, single engine light private airplane. The characteristics of the airplane are as follows: aspect ratio = 6.2, wing area = 181 ft.2, Oswald efficiency factor = 0.91, weight = 3000 lb, and parasite drag coefficient = 0.027. The airplane is powered by a single piston engine of 345 hp maximum at sea level. Assume the power of the engine is proportional to free stream density. The two-bladed propeller has an efficiency of 0.83.Β
- Calculate the power required at sea level.
- Calculate the maximum velocity at sea level.
- Calculate the power required at 12,000 ft. altitude.
- Calculate the maximum velocity at 12,000 ft. altitude.

Consider an airplane patterned after the twin-engine Beechcraft Queen Air executive transport. The airplane weight is 38,220 N, wing area is 27.3 m2, aspect ratio is 7.5. Oswald efficiency factor is 0.9 and parasite drag coefficient CDo = 0.03. Calculate the thrust required to fly at a velocity of 350 km/h at (a) standard sea level and (b) an altitude of 4.5 km.
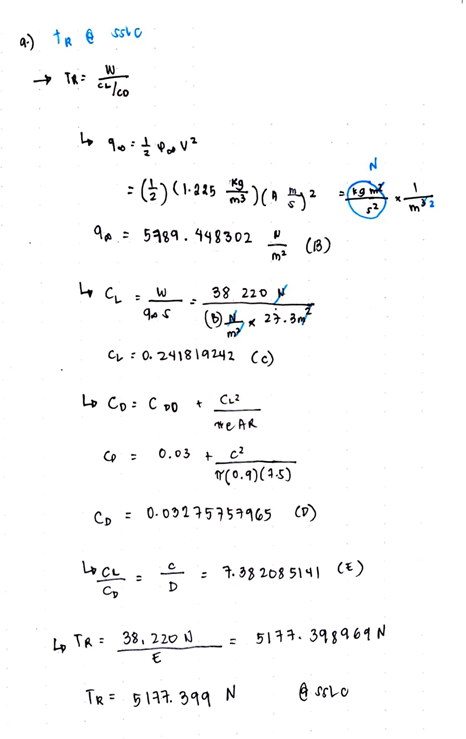
Chemical energy | Reciprocating engines operate on the basic principle of converting ___ (fuel) into mechanical energy. |
Combustion | Chemical to mechanical energy |
2 primary reciprocating engine designs | Spark and compression ignition |
Compression engine | Also known as jet fuel piston engine |
Compression ignition engines | Have the added advantage of utilizing readily available and lower cost diesel or jet fuel |
Similarities of spark ignition and compression ignition engine | They use cylindrical combustion chambers and pistons that travel the length of the cylinders to convert linear motion into the rotary motion of the crankshaft. |
Difference between spark ignition and compression ignition engine | Process of igniting the fuel |
Spark ignition engines | Use a spark plug to ignite a pre-mixed fuel-air mixture. |
Compression engines | First compresses the air in the cylinder, raising its temperature to a degree necessary for automatic ignition when fuel is injected into the cylinder. |
Types of radial engine | Rotary and static type |
Inline Engines | Have an even number of cylinders aligned in a single row parallel with the crankshaft |
Inline engines | These engines have a comparatively small frontal area, which enables them to be enclosed by streamlined nacelles or cow lings |
Banks | In V-type engines, Two rows of cylinders, called ___, are oriented 45, 60, or 90 degrees apart from a single crankshaft. |
in-line engine | In V-type engine, two banks of cylinders typically produce more horsepower than an ___. |
Opposed-type engines | Most common reciprocating engines currently used on light aircraft |
Opposed engines | These engines have a relatively small, lightweight crankcase that contributes to a high power to-weight ratio. |
Cylinder assembly | The valves, pistons, and spark plugs are located in the ___. |
Crankcase | The valve operating mechanism, crankshaft, and connecting rods are located in the ___. |
Crankcase | Core of a reciprocating engine. It contains the engine’s internal parts and provides attach points for the cylinders, external accessories, and airframe installation. |
Crankcase | Provides a tight enclosure for the lubricating oil |
Cylinder | The combustion chamber where the burning and expansion of gases takes place to produce engine power |
Piston | A cylindrical plunger that moves up and down within a cylinder assembly |
Piston | It manages the fuel, air, and exhaust pressures in the cylinder. |
Piston | It transmits the force of combustion through the connecting rod to the crankshaft. |
Connecting rods | The link that transmits the force exerted on the piston to the crankshaft. |
Valves | Engine valves regulate the flow of gases into and out of a cylinder by opening and closing at the appropriate time during the Otto cycle. |
Crankshaft | It receives a linear power pulse from the piston through the connecting rod and changes it to rotary motion to turn the propeller. |
Operating principles | |
Internal combustion engine | Because the fuel is burned inside the engine, an aircraft engine is referred to as an ___. |
Nitrogen | The fuel/air mixture is compressed and ignited within a cylinder. As the mixture burns, it releases energy, causing the noncombustible gases, like ___, to expand. |
5 events of engine combustion in reciprocating engine (ICIPE) | Intake, compression, ignition, power, exhaust |
4 strokes of Otto Cycle (ICPE) | Intake, compression, power, exhaust |
2 | Completing a four-stroke cycle requires ___ revolutions of the crankshaft. |
Constant volume cycle | The four-stroke cycle is sometimes referred to as a ___ because the burning fuel inside the cylinder increases pressure with almost no change in volume. |
Compression | The ___ stroke begins when the intake valve closes, and the piston starts moving back to the top of the cylinder. This phase of the cycle is used to obtain a much greater power output from the fuel-air mixture once it is ignited. |
Power | The ___ stroke begins when the fuel-air mixture is ignited. This causes a tremendous pressure increase in the cylinder and forces the piston downward away from the cylinder head, creating the power that turns the crankshaft. |
Work | The result of force moving an object. |
Time | It is not a consideration when determining the amount of work done. |
Power | Work related to time or the time-rate of doing work |
Fd/T | Formula of power |
1 Hp | The amount of power required to do 33,000 foot-pounds of work in one minute or 550 footpounds of work in one second. |
F d/ 33 000 t | Formula for calculating horsepower |
Indicated horsepower (IHP) | The total power developed in the cylinders without accounting for friction losses within the engine. |
Indicated mean effective pressure (IMEP) | To calculate indicated horsepower, you must know the ___ within the cylinders. |
IHP = PLANK/33 000 | Formula of indicated horsepower (IHP) |
In IHP = PLANK/33 000, what is P? | Indicated mean effective pressure (IMEP) |
In IHP = PLANK/33 000, what is L? | The length of the stroke in feet |
In IHP = PLANK/33 000, what is N? | The number of power strokes per minute for one cylinder |
In IHP = PLANK/33 000, what is K? | The number of cylinders |
Friction horsepower | The power necessary to overcome the friction and energy losses |
Brake horsepower | The actual amount of power delivered to turn a propeller |
Brake horsepower | Friction HP – IMP |
Brake horsepower | It involves measuring torque, or twisting moment. |
2π π₯ πππππ’π π₯ πππ/ 33 000 | Brake horsepower |
Parts of aircraft turbine engine | Air inlet, compressor, combustion chambers, turbine section, and exhaust. |
Thrust | It is produced by increasing the velocity of the air flowing through the engine. |
Turbine | It turns energy from the working fluid to rotational motion |
Turbojet vs Turbofan | Turbofan has fan, HP/LP shaft and turbine, and by-pass air |
Turboprop | This jet engine has gearbox and propeller |
Turboshaft | This jet engine has a power shaft |
Turbojet | This engine consists of four sectionsβcompressor, combustion chamber, turbine section, and exhaust. |
Turbojet | These engines are limited in range and endurance. They are also slow to respond to throttle applications at slow compressor speeds. |
Turboprop | An engine is a turbine engine that drives a propeller through a reduction gear. |
Reduction gearing | It is necessary in turboprop engines because optimum propeller performance is achieved at much slower speeds than the engineβs operating rpm. |
Turbofan | Combined features of turbojet and turboprop. These engines are designed to create additional thrust by diverting a secondary airflow around the combustion chamber. |
Bypass air | Generates increased thrust, cools the engine, and aids in exhaust noise suppression. |
Turboshaft | It delivers power to a shaft that drives something other than a propeller. |
Turbojet vs Turboshaft | On a turboshaft engine, most of the energy produced by the expanding gases is used to drive a turbine rather than produce thrust. |
Turboshaft | These engines are widely used as auxiliary power units on large aircraft. |
Engine components β cold section | Air inlet and compressor |
Engine components β hot section | Combustors, turbines, and exhaust |
Air inlet duct | It provides a uniform supply of air to the compressor for efficient operation. |
Gas turbine engine | It takes in a quantity of air, adds energy to it, and then discharges the air to produce thrust. |
Compressor | The component forcing air into an engine |
Combustion section | It contains the same basic elements: one or more combustion chambers (combustors), a fuel injection system, an ignition source, and a fuel drainage system. |
Combustion section | This is where fuel and air is mixed. |
Turbine | It transforms a portion of the kinetic energy in the hot exhaust gases into mechanical energy to drive the compressor and accessories. |
4 basic elements of a turbine section (CSSR) | Case, stator, shroud, and rotor |
Exhaust section | It is where the exhaust gases leave the engine to produce thrust. |
Parts of exhaust section | Exhaust duct or tailpipe, and exhaust nozzle |
Brayton cycle | The energy transformation cycle in a gas turbine engine |
Brayton cycle | Also known as constant pressure cycle |
Brayton cycle | Similar to the four-stroke Otto cycle, the Brayton cycle has intake, compression, combustion, and exhaust events. |
Gas turbine engine | Unlike a piston engine, all four events occur simultaneously and continuously in a gas turbine engine. A __ is able to produce power continuously. |
Thrust | The propeller is a case in point, where the net result of the pressure and shear stress distributions over the surface of the propeller blades yields this aerodynamic force, which propels the vehicle forward. |
Jet engine | Takes air (at free stream velocity)> heat/combust > exhaust = thrust |
Jet engine | It creates a change in momentum of the gas by taking a small mass of air and giving it a large increase in velocity. |
Fundamental source of the thrust of a jet engine | The NET FORCE produced by the pressure and SHEAR STRESS distributions exerted over the surface of the engine |
General formula for thrust for jet engines | T = mair (Ve β Vβ) + (Pe β Pβ) Ae |
Reciprocating engine | Similar to Otto cycle |
Gas turbine engine | Similar to Brayton cycle (continuous power) |
Momentum thrust | Thrust produced by change in momentum |
Pressure thrust | Thrust produced by change in momentum produce by chance in Pressure |
Sources of aerodynamic forces | Pressure and shear stress distribution |
Pressure and shear stress distribution | Aerodynamic forces and moments exerted on a body moving through a fluid came from two sources which acts over the body surface: ___ |
Drag polar equation | |
Drag polar equation | Includes the drag coefficient for the whole plane |
C DE | Parasite drag coefficient (profile, skin friction, and pressure drag) |
Wave drag | At transonic and supersonic speeds, the parasite drag coefficient also contains ___. |
Wave drag | This drag can be found in supersonic conditions only |
Skin friction drag | Drag due to SHEAR STRESS |
Pressure drag | Drag due to FLOW SEPARATION |
Pressure drag | Also known as form or profile drag |
Induced drag | Drag produced by wing tip vortices or downwash |
3 main drags | Skin friction, pressure, and induced drag |
Drags due to flow separation | Par, Pre, Pro, Fo: Parasite, Pressure, Profile, Form drag |
Parasite drag | Also known as profile drag |
Drag divergence mach number | It is where the onset of drag increase is imminent due to the transition to supersonic flows and that for supersonic flows. Clue: Wave drag is noticeable here |
Wave drag | Caued by the difference in pressure ahead and behind the shockwave which gives a net effect in the direction of the greater pressure acting in the drag direction. |
Wave drag | Drag needed to be overcome by the aircraft powerplant. |
Interference drag | A drag generated from the mixing of airflow streamlines between airframe components which causes eddies. |
Span efficiency factor (e) | It is accounting for non-elliptical wing planforms. Also known as Oswaldβs Efficiency Factor. |
Lift | Perpendicular to the flight path direction |
Drag | Parallel to the flight path direction |
Weight | Acts vertically toward the center of the earth (and hence is inclined at an angle Ο΄ with respect to the lift direction) |
Thrust | Inclined at an angle ππ with respect to the flight path direction |
Curvilinear motion | An object that moves along a curved path |
Rectilinear motion | An object that follows a straight line |
Translational flight | It describes the general two dimensional motion of an airplane in an accelerated flight. |
Static performance | Unaccelerated flight conditions of an airplane |
(L/D) ratio is a maximum | The value of TR depends inversely as the L/D ratio and, thus the minimum thrust required (TRmin) will be obtained when the airplane is flying at a velocity where the lift to drag ___. |
(L/D)max | Tr min (minimum thrust) is equal to⦠|
Why (L/D)max is important? | L/Dmax is the point of minimum drag, and is where the best glide speed of the airplane occurs. No other angle of attack will give you lower drag for the lift required. |
High Tr and High Vβ | Based on the TR vs π½β of an Airplane diagram, if the dynamic pressure (q) is high, the velocity is also high. |
Inversely proportional | Most of the required lift by the aircraft at a point on TR vs π½β of an Airplane diagram (High Tr & High V free stream) is obtained by the high dynamic pressure (πβ) and since it is ___ to lift coefficient (CL), the value of angle of attack (π) should be small, so as to produce a small value of the lift coefficient (CL). CLUE: L = S q Cl |
Angle of attack (π) | The value of ___ should be small, so as to produce a small value of the lift coefficient (CL) based on the TR vs π½β of an Airplane diagram. |
Dynamic pressure (πβ) | Drag is relatively large because the ___ is large in value based on the TR vs π½β of an Airplane diagram. |
Relationship of drag to the TR vs π½β of an Airplane diagram. | High drag = high dynamic pressure (πβ) |
Decreases | Dynamic pressure (πβ) __, drag and thrust required (TR) in turn decrease initially from point (a) to lower values of velocity (πβ), based on the TR vs π½β of an Airplane diagram. |
q S C do | Parasite thrust required |
q S (Cl2/pi eAR) | Induced thrust required |
increase | Induced thrust required is decreasing with ___ in velocity and that the parasite thrust required is increasing with increase in velocity, based on the TR vs π½β of an Airplane diagram. |
Why is the coefficient of lift is decreasing with increase in velocity? | Based on the TR vs π½β of an Airplane diagram, since the presence of dynamic pressure πβ is high enough to create majority of the lift and since the coefficient of lift is small, the induced thrust required consequently decreases. |
increases | If the dynamic pressure πβ is high, the parasite drag ___. |
Parasite drag | A combination of profile (form) drag, skin friction drag, and interference drag |
induced drag | At TRmin conditions, the parasite drag is equal to the ___ as shown by their respective coefficients. This was shown by the graph (Breakdown of TR vs π½βof an Airplane), since the point of intersection of the parasite thrust required and induced thrust required intersected at a point corresponding to velocity for TRmin where the lift to drag (L/D) ratio is a maximum. |
maximum | The thrust available produced by a piston engine with propellers is a ___ at zero velocity (static thrust) and it decreases with forward velocity. |
Static thrust | Maximum at zero velocity |
Constant | The jet engine produces a relatively ___ thrust with respect to its velocity. |
Ta (Thrust A) | Produced by engines |
Tr (Thrust R) | Dictated by the aerodynamics and weight of the airplane which is an airframe-associated phenomenon |
Thrust | Conventional jet engines are rated in terms of ___. Usually in lbs. |
Horsepower | Piston engines are rated in terms of ___. |
TA and TR | They are inconvenient for propeller driven aircrafts because jet engines are rated in terms of thrust and piston engines are rated in HP. Power required and power available are used for propeller driven aircrafts. |
Power | Energy/time |
inversely | Thrust required varies ___ to the lift to drag ratio |
Power required curve | It is defined as a plot of the Power Required (PR) versus velocity () |
Power required curve | It resembles the thrust required curve in a sense that the power required will decrease, hit a minimum and increase again. |
Also increases | Based on the power required curve, the power will increase if the velocity free stream ___. |
lift to drag ratio | Based on the power required curve, the minimum point corresponds to the maximum ___ |
( | The conditions for minimum power required is given by the slope of the equation of the power required curve. |
The point 1 in the figure means⦠| It corresponds to CDo = CDi that is minimum TR, hence the velocity for minimum PR is less than that for the minimum TR. |
Shaft brake power (P) | The power delivered to the propeller by the crankshaft |
Brake stems | Measures engine power by loading it with calibrated brake mechanisms |
ππ΄ = β΄π | Power available |
ππ΄ = β΄π, what is n? | Propeller efficiency which is less than 1 |
ππ΄ = β΄π, what is P? | Shaft break power |
550 ft lb/s = 746 watts | 1 HP is equals to⦠|
βππ΄ = β΄β π΅π»π | Power available |
increase | The power available curve for a piston engine and propeller combination shows that the ___ in power = increase in V free stream |
Jet engine | Derives its thrust by combustion heating an incoming stream of air and then exhausting this hot air at high velocities through a nozzle |
Effects of Altitude for Power Required | Pr is proportional to V freestream |
Power available | The ___ for altitudes experiences a reduction in power because of low air density at that altitudes. |
An aircraft at an altitude would stall beyond the minimum velocity because? | From the intersection of the PA and PR, figure 1.19 shows that at an altitude, the minimum velocity can be greater than the actual stalling velocity. |